Abstract
Caffeine, a widely consumed psychoactive substance, plays a complex role in human physiology, often seen as both a beneficial ally and a potential adversary. This abstract explores the dual nature of caffeine's influence on the body. As a stimulant, caffeine enhances alertness, improves cognitive performance, and elevates mood by blocking adenosine receptors in the brain. It can also increase physical endurance and may provide protective effects against neurodegenerative diseases and certain metabolic disorders. However, excessive caffeine intake can lead to negative consequences, including anxiety, insomnia, and increased heart rate. For sensitive individuals, even moderate consumption may trigger adverse effects. Furthermore, dependence on caffeine can result in withdrawal symptoms, complicating its role in daily life. This paper evaluates current research on caffeine's pharmacological effects, its impact on mental and physical health, and the balance between its benefits and drawbacks. Ultimately, the classification of caffeine as a friend or foe hinges on individual tolerance, consumption patterns, and overall lifestyle. By understanding these dynamics, we can make informed choices regarding caffeine intake and its implications for our health.
Keywords
Caffeine, Stimulant, Alertness, Dependence, Health, Tolerance
Introduction
Caffeine, a naturally occurring stimulant found in various plants, is one of the most widely consumed substances globally. It is primarily recognized for its ability to enhance alertness and combat fatigue, making it a popular choice in beverages such as coffee, tea, and energy drinks. The physiological effects of caffeine stem from its action on the central nervous system, where it blocks adenosine receptors, leading to increased neuronal firing and the release of neurotransmitters like dopamine and norepinephrine. While moderate caffeine consumption can offer several benefits, including improved cognitive function and physical performance, the narrative surrounding caffeine is not entirely positive. Overconsumption can lead to a range of negative health effects, such as anxiety, insomnia, and heart palpitations. Furthermore, individuals can develop a dependence on caffeine, experiencing withdrawal symptoms when intake is reduced.
Given these contrasting effects, the question of whether caffeine serves as a friend or foe is multifaceted. Individual tolerance, lifestyle factors, and personal health conditions significantly influence caffeine's impact. This introduction sets the stage for a deeper exploration of caffeine’s role in human functioning, weighing its potential benefits against its drawbacks, and ultimately aiming to provide a nuanced understanding of its effects on health and well-being. By analyzing current research, we can better appreciate caffeine’s dual nature and make informed decisions regarding its consumption.
CAFFEINE - GENERAL INFORMATION
Caffeine (1,3,7-trimethylcanthine or 3,7-dihydro-1,3,7-trimethyl-1H-purine-2,6-dione), a well-known purine alkaloid, was described by Gennaro [8] as a white, odorless powder with a slightly bitter taste. Its chemical formula is C8H10N4O2. Caffeine occurs in more than 60 plant species globally. Caffeine is a central nervous system stimulant found in coffee, tea, chocolate, and various energy drinks. It primarily works by blocking adenosine receptors in the brain, which helps increase alertness and reduce fatigue. Caffeine is also associated with enhanced cognitive performance and improved physical endurance. While moderate consumption can offer benefits, excessive intake may lead to negative effects such as anxiety, insomnia, and increased heart rate. Individual tolerance varies, and some people may experience dependence, leading to withdrawal symptoms upon cessation. Understanding caffeine's effects is essential for making informed choices about its consumption.
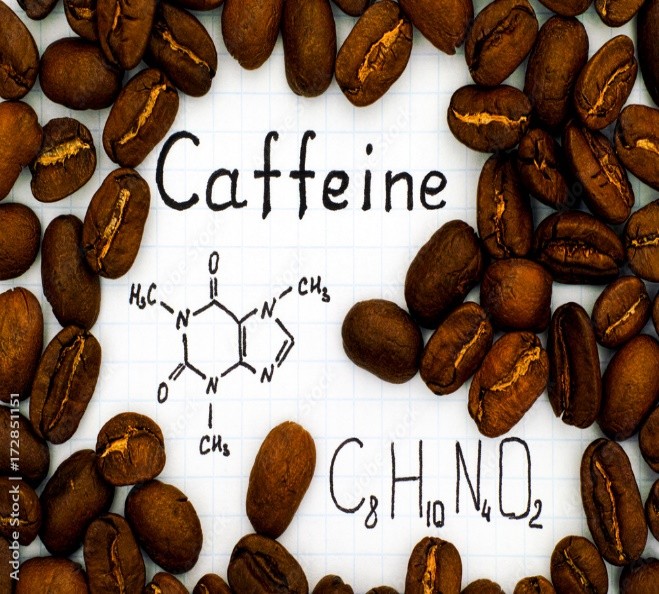
Fig:1 Chemical formula and structure of caffeine
METABOLISM OF CAFFEINE
Caffeine metabolism occurs primarily in the liver, where it is broken down by enzymes, mainly cytochrome P450 1A2. This process transforms caffeine into three primary metabolites: paraxanthine, theobromine, and theophylline, each of which has distinct effects on the body. Paraxanthine is known for its role in enhancing lipolysis, promoting the breakdown of fats for energy. Theobromine, found in chocolate, has mild stimulant effects and can also contribute to improved mood and cardiovascular function. Theophylline, used in some medical treatments, can relax bronchial muscles and improve breathing.
The rate of caffeine metabolism varies significantly among individuals due to genetic factors, lifestyle choices, and certain medications. For example, genetic polymorphisms in the CYP1A2 gene can result in "fast" or "slow" metabolizers. Fast metabolizers process caffeine quickly, often experiencing less pronounced effects and requiring higher doses to achieve similar benefits. In contrast, slow metabolizers may feel the effects more intensely and for longer periods, increasing the risk of side effects such as anxiety or insomnia.
Factors such as age, pregnancy, and overall health also influence metabolism rates. Pregnant individuals, for instance, often metabolize caffeine more slowly, prompting recommendations to limit intake during pregnancy. Additionally, habitual caffeine consumption can lead to tolerance, where the body adjusts to its presence, altering its effects. Understanding caffeine metabolism is crucial for managing its consumption and recognizing individual responses, helping individuals make informed choices about their caffeine intake.
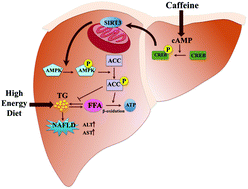
Fig:2 Pathway of caffeine metabolism in liver
GENETICS AND CAFFEINE
Genetics significantly influences how individuals metabolize caffeine and respond to its effects. The primary enzyme involved in caffeine metabolism is cytochrome P450 1A2, encoded by the CYP1A2 gene. Variations in this gene can classify individuals as "fast" or "slow" metabolizers. Fast metabolizers process caffeine quickly, often experiencing milder effects and requiring higher doses to achieve the same stimulation as slow metabolizers, who may feel pronounced effects from smaller amounts.
Research indicates that slow metabolizers are more susceptible to adverse reactions, such as increased anxiety, insomnia, and elevated heart rate, particularly with high caffeine consumption. Additionally, genetic factors can affect caffeine's impact on mood and cognitive function. Certain genetic variants may enhance the cognitive benefits of caffeine, improving focus and alertness for some, while others may experience diminished effects.
Other genetic elements, including variations in adenosine receptors, also play a role in caffeine sensitivity. These receptors are the primary targets of caffeine in the brain, and genetic differences can influence how caffeine interacts with them. For instance, individuals with specific receptor variants may find caffeine more effective at improving performance or mood.
Furthermore, genetics can interact with lifestyle factors, such as diet and overall health, to shape individual responses to caffeine. For example, heavy smokers metabolize caffeine differently, which can further complicate the relationship between genetics and caffeine effects. Understanding these genetic influences is vital for tailoring caffeine consumption to individual needs, enabling people to optimize its benefits while minimizing risks. As research advances, personalized recommendations based on genetic profiles could become an essential aspect of dietary guidance regarding caffeine.
EFFECTS ON RECEPTORS
Caffeine exerts its effects primarily through its interaction with adenosine receptors in the brain. Adenosine is a neurotransmitter that promotes relaxation and sleepiness. By blocking adenosine receptors, particularly the A1 and A2A subtypes, caffeine reduces the feeling of fatigue and enhances alertness. This inhibition leads to increased neuronal activity and the release of other neurotransmitters, such as dopamine and norepinephrine, contributing to improved mood and cognitive performance. Additionally, caffeine can influence dopamine receptors, enhancing the pleasure and reward pathways in the brain, which is why many people find caffeine stimulating and enjoyable. However, excessive caffeine intake can lead to overstimulation of these receptors, resulting in anxiety, restlessness, and disrupted sleep patterns. Understanding caffeine's effects on these receptors helps explain its dual nature as both a potential cognitive enhancer and a source of adverse reactions, depending on individual sensitivity and consumption levels.
TOXICITY OF CAFFEINE
Caffeine toxicity occurs when excessive amounts are consumed, leading to adverse health effects. Common symptoms include restlessness, insomnia, headaches, dizziness, and increased heart rate. Severe cases can result in more serious issues, such as cardiac arrhythmias, seizures, or even life-threatening situations. The median lethal dose of caffeine is estimated to be around 10 grams for most adults, which is equivalent to consuming about 100 cups of coffee in a short time frame. Individual tolerance varies significantly based on factors such as genetics, age, and overall health. Pregnant individuals and those with certain medical conditions should limit their intake to avoid potential risks. Understanding caffeine's toxicity is essential for safe consumption and overall health.
ADENOSINE
Adenosine is a naturally occurring neurotransmitter that plays a crucial role in promoting sleep and relaxation. Throughout the day, adenosine levels gradually increase in the brain, leading to a sense of tiredness and the eventual need for sleep. Caffeine interferes with this process by blocking adenosine receptors, specifically the A1 and A2A subtypes. By doing so, caffeine prevents adenosine from exerting its calming effects, resulting in heightened alertness and reduced fatigue.
This blockade also stimulates the release of other neurotransmitters, such as dopamine and norepinephrine, enhancing mood and cognitive function. However, excessive caffeine consumption can lead to overstimulation, resulting in anxiety, restlessness, and disrupted sleep patterns. Over time, regular caffeine use can lead to tolerance, as the body adjusts to its presence, requiring higher doses to achieve the same stimulating effects. Understanding the role of adenosine in the body helps clarify why caffeine can be both a beneficial ally in enhancing alertness and a potential foe when consumed in excess.
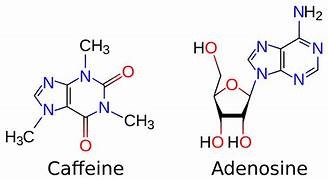
Fig:3 The comparison of caffeine and adenosine
THE ROLE OF CAFFEINE IN VARIOUS SYSTEMS IN THE HUMAN BODY
Caffeine is a widely consumed psychoactive substance known for its stimulating effects. Found in coffee, tea, chocolate, and various energy drinks, it has significant impacts on multiple systems within the human body. Understanding how caffeine interacts with different physiological systems helps clarify its dual role as both a potential ally and a source of adverse effects.
1. CENTRAL NERVOUS SYSTEM (CNS)
Caffeine's primary action occurs in the central nervous system, where it functions as a stimulant. By blocking adenosine receptors, particularly A1 and A2A, caffeine reduces feelings of fatigue and increases alertness. This mechanism not only enhances focus and cognitive performance but also elevates mood by promoting the release of neurotransmitters like dopamine and norepinephrine.
Research indicates that moderate caffeine consumption can improve attention, reaction times, and even short-term memory. However, excessive intake can lead to negative outcomes, such as anxiety, insomnia, and jitteriness. Over time, regular use can result in tolerance, where the initial stimulating effects diminish, requiring higher doses to achieve the same impact.
2. CARDIOVASCULAR SYSTEM
Caffeine has complex effects on the cardiovascular system. Initially, it can increase heart rate and blood pressure, primarily due to its stimulating effects on the heart and its ability to increase catecholamine release. For most healthy individuals, moderate caffeine intake is not associated with long-term cardiovascular risk and may even offer protective benefits against certain heart conditions.
However, sensitivity varies among individuals. Some people may experience palpitations or elevated blood pressure with even small amounts of caffeine. For those with pre-existing heart conditions, moderation is crucial, as excessive caffeine consumption can exacerbate these issues.
3. MUSCULAR SYSTEM
Caffeine is also known to enhance physical performance, making it a popular ergogenic aid among athletes. It increases endurance and reduces the perception of effort during physical activities. By stimulating the release of calcium from the sarcoplasmic reticulum in muscle cells, caffeine can enhance muscle contractions, leading to improved performance in high-intensity and endurance exercises.
Furthermore, caffeine encourages fat oxidation, promoting the use of fat as a fuel source during exercise. This effect can be particularly beneficial for endurance athletes, allowing for prolonged activity without depleting glycogen stores. However, individual responses to caffeine can vary, with some experiencing gastrointestinal distress or nervousness during physical exertion.
4. METABOLIC SYSTEM
Caffeine affects the metabolic system by increasing the metabolic rate and promoting fat oxidation. It stimulates thermogenesis, the process by which the body produces heat and energy from digesting food. Studies have shown that caffeine can temporarily boost metabolism by about 3-11%, depending on individual factors such as body composition and habitual caffeine consumption.
Moreover, caffeine may have potential implications for weight management. By enhancing fat breakdown and improving exercise performance, it can contribute to weight loss efforts. However, reliance on caffeine alone for weight management is not advisable, as long-term effects on weight and health require a balanced diet and regular physical activity.
5. GASTROINTESTINAL SYSTEM
Caffeine's impact on the gastrointestinal system is multifaceted. It can stimulate gastric acid secretion, which may aid in digestion but can also lead to discomfort or exacerbate conditions like gastroesophageal reflux disease (GERD). In some individuals, caffeine can act as a laxative, promoting bowel movements.
The effects on gut health are less straightforward. While moderate caffeine intake is generally considered safe for most, excessive consumption can lead to gastrointestinal disturbances, such as nausea and diarrhea. For those with sensitivities, limiting caffeine may be necessary to avoid discomfort.
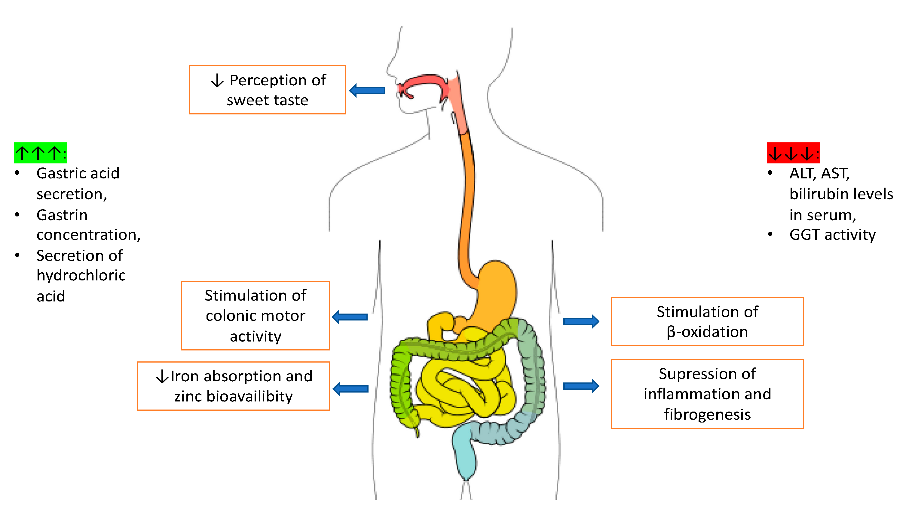
Fig:4 The scheme of the main effects of caffeine on gastrointestinal syestem
6. ENDOCRINE SYSTEM
Caffeine influences the endocrine system by affecting hormone levels, particularly cortisol and adrenaline. It stimulates the adrenal glands, leading to increased secretion of adrenaline, which can enhance alertness and physical performance. However, chronic caffeine consumption can lead to elevated cortisol levels, potentially affecting stress responses and overall hormonal balance.
Additionally, caffeine may influence insulin sensitivity. Some studies suggest that moderate caffeine consumption may improve insulin sensitivity, while excessive intake may have the opposite effect. This interaction highlights the importance of moderation, especially for individuals with insulin resistance or diabetes.
7. RENAL SYSTEM
Caffeine is known to have diuretic properties, promoting increased urine production. This effect can be beneficial for hydration in moderate amounts, but excessive caffeine can lead to dehydration, especially during intense physical activity. The renal system’s response to caffeine can vary; regular consumers may develop a tolerance to its diuretic effects.
While caffeine can influence kidney function, moderate consumption is generally considered safe for healthy individuals. However, those with pre-existing kidney issues should consult healthcare providers regarding their caffeine intake, as excessive consumption may pose risks.
8. PSYCHOLOGICAL AND BEHAVIORAL EFFECTS
Caffeine can significantly affect psychological states and behavior. In moderate doses, it is associated with enhanced mood, alertness, and cognitive function. However, high doses can lead to anxiety, irritability, and sleep disturbances. Individuals sensitive to caffeine may experience heightened negative effects even with low to moderate consumption.
The relationship between caffeine and mental health is complex. Some studies suggest that moderate caffeine consumption may be linked to a lower risk of depression and neurodegenerative diseases like Alzheimer’s and Parkinson’s. However, excessive intake could contribute to anxiety disorders and sleep disturbances, underscoring the need for balance.
In conclusion caffeine's role in the human body is multifaceted, influencing various systems from the central nervous system to the cardiovascular and metabolic systems. While moderate consumption can enhance cognitive function, physical performance, and mood, excessive intake may lead to adverse effects and health risks. Individual responses to caffeine can vary widely due to genetic factors, tolerance levels, and overall health conditions. Understanding the complexities of caffeine's effects is essential for making informed choices regarding its consumption. By balancing the potential benefits and drawbacks, individuals can harness caffeine's positive effects while minimizing risks, ultimately enhancing their well-being.
THE RELATIONSHIP BETWEEN CAFFEINE AND OXIDATIVE STRESS
Caffeine, a ubiquitous stimulant found in coffee, tea, and various energy drinks, has garnered significant attention for its effects on human health, particularly concerning oxidative stress. Oxidative stress occurs when there’s an imbalance between free radicals and antioxidants in the body, leading to cellular damage and contributing to various diseases, including cardiovascular disorders, neurodegenerative diseases, and cancer. Understanding the role of caffeine in this context reveals both potential benefits and risk.
CAFFEINE AS AN ANTIOXIDANT
Research suggests that caffeine exhibits antioxidant properties, which can help mitigate oxidative stress. Antioxidants neutralize free radicals, thereby protecting cells from damage. Studies have indicated that caffeine can enhance the body’s antioxidant defenses by upregulating the expression of certain antioxidant enzymes. This action can contribute to a protective effect against oxidative stress-related damage, particularly in the context of chronic diseases.
Moreover, caffeine’s ability to promote the production of other antioxidants in the body, such as glutathione, adds to its protective profile. This can be particularly beneficial in preventing oxidative damage in organs like the liver and brain, which are often susceptible to the harmful effects of free radicals.
POTENTIAL RISKS OF EXCESSIVE CAFFEINE
While moderate caffeine consumption may confer protective benefits against oxidative stress, excessive intake can have the opposite effect. High doses of caffeine can lead to increased production of reactive oxygen species (ROS), which are harmful free radicals. This paradox highlights the importance of moderation. In individuals with preexisting conditions or those consuming caffeine in excessive amounts, the balance can shift toward oxidative stress, potentially exacerbating health issues.
Furthermore, caffeine can stimulate the central nervous system, leading to increased metabolic activity. This heightened activity may also generate more free radicals, contributing to oxidative stress if the body’s antioxidant defenses are overwhelmed.
IMPLICATIONS FOR HEALTH
The relationship between caffeine, oxidative stress, and health outcomes is complex. Moderate caffeine consumption has been associated with reduced risks of certain conditions, including neurodegenerative diseases like Parkinson’s and Alzheimer’s. The antioxidant properties of caffeine may play a role in this protective effect, helping to combat oxidative stress in the brain.
However, individuals with certain health conditions, such as anxiety disorders or cardiovascular issues, should approach caffeine with caution. For these populations, the potential for increased oxidative stress from excessive caffeine intake could pose significant health risks.
THE ASSOCIATION BETWEEN CAFFEINE CONSUMPTION AND MORTALITY
Caffeine, widely consumed through coffee, tea, and energy drinks, has been the subject of extensive research regarding its impact on health, particularly concerning mortality rates. Studies examining the relationship between caffeine consumption and all-cause and cause-specific mortality have yielded intriguing findings, suggesting both protective and neutral effects.
ALL-CAUSE MORTALITY
Several large-scale epidemiological studies have indicated that moderate caffeine consumption is associated with a reduced risk of all-cause mortality. For instance, research suggests that individuals who consume 3 to 4 cups of coffee per day may experience lower mortality rates compared to non-coffee drinkers. The potential mechanisms behind this protective effect include caffeine's antioxidant properties, anti-inflammatory effects, and its ability to improve metabolic health.
CAUSE-SPECIFIC MORTALITY
When examining cause-specific mortality, the associations become more nuanced. For cardiovascular health, moderate caffeine intake has been linked to a lower risk of heart disease and stroke. Conversely, excessive caffeine consumption, particularly among sensitive individuals, may lead to increased heart rate and hypertension, potentially raising mortality risk in these groups.
In the context of cancer, findings are mixed. Some studies suggest that caffeine may offer protective benefits against certain types of cancer, such as liver and colorectal cancers. However, other types may not show the same association, indicating that the relationship is complex and influenced by various factors, including genetics and lifestyle.
CONCLUSION
Caffeine's role in the human body is multifaceted, acting as both a friend and a foe depending on the context of its consumption. As a widely consumed stimulant, caffeine offers various benefits, including enhanced alertness, improved cognitive performance, and potential protective effects against certain diseases, such as neurodegenerative disorders and some forms of cancer. Moderate intake is often linked to reduced risks of all-cause mortality and specific health conditions, making it a potentially valuable component of a healthy lifestyle. However, excessive caffeine consumption can lead to adverse effects, including increased heart rate, elevated blood pressure, and heightened anxiety. For individuals with certain health conditions, the risks may outweigh the benefits, emphasizing the importance of personalized consumption. Additionally, factors such as tolerance and dependence can complicate caffeine's effects on well-being. Ultimately, understanding caffeine's dual nature is essential for making informed choices. Moderation is key, allowing individuals to enjoy the benefits while minimizing potential harm. By considering personal health status, tolerance, and lifestyle factors, individuals can navigate caffeine consumption effectively. As research continues to evolve, staying informed about the latest findings will help people make better decisions regarding their caffeine intake, ensuring it serves as a friend rather than a foe in their overall health journey.
REFERENCE
- Mahoney C.R., Giles G.E., Marriott B.P., Judelson D.A., Glickman E.L., Geiselman P.J., Lieberman H.R. Intake of caffeine from all sources and reasons for use by college students. Clin. Nutr. 2019;38:668–675. doi: 10.1016/j.clnu.2018.04.004. [PubMed] [CrossRef] [Google Scholar]
- Heckman M.A., Weil J., De Mejia E.G. Caffeine (1, 3, 7-trimethylxanthine) in Foods: A Comprehensive Review on Consumption, Functionality, Safety, and Regulatory Matters. J. Food Sci. 2010;75:R77–R87. doi: 10.1111/j.1750-3841.2010.01561.x. [PubMed] [CrossRef] [Google Scholar]
- Chawla J. Neurologic Effects of Caffeine Physiologic Effects of Caffeine. Medscape Ref. Drug Dis. Proced. 2015;24:1–8. [Google Scholar]
- Conway J. Global Coffee Consumption, 2020/21|Statista. [(accessed on 5 May 2021)]. Available online: https://www.statista.com/statistics/292595/global-coffee-consumption/
- Satel S. Is Caffeine Addictive?—A Review of the Literature. Am. J. Drug Alcohol Abus. 2006;32:493–502. doi: 10.1080/00952990600918965. [PubMed] [CrossRef] [Google Scholar]
- Kolahdouzan M., Hamadeh M.J. The neuroprotective effects of caffeine in neurodegenerative diseases. CNS Neurosci. Ther. 2017;23:272–290. doi: 10.1111/cns.12684. [PMC free article] [PubMed] [CrossRef] [Google Scholar]
- Pohanka M., Dobes P. Caffeine Inhibits Acetylcholinesterase, But Not Butyrylcholinesterase. Int. J. Mol. Sci. 2013;14:9873–9882. doi: 10.3390/ijms14059873. [PMC free article] [PubMed] [CrossRef] [Google Scholar]
- IARC Coffee, tea, mate, methylxanthines and methylglyoxal. IARC Monogr. Eval. Carcinog. Risks Hum. 1991;51:1–513. [PMC free article] [PubMed] [Google Scholar]
- Barone J.J., Roberts H. Caffeine. Springer; Berlin/Heidelberg, Germany: 1984. Human Consumption of Caffeine; pp. 59–73. [Google Scholar]
- De Mejia E.G., Ramirez-Mares M.V. Impact of caffeine and coffee on our health. Trends Endocrinol. Metab. 2014;25:489–492. doi: 10.1016/j.tem.2014.07.003. [PubMed] [CrossRef] [Google Scholar]
- Blanchard J., Sawers S.J.A. The absolute bioavailability of caffeine in man. Eur. J. Clin. Pharmacol. 1983;24:93–98. doi: 10.1007/BF00613933. [PubMed] [CrossRef] [Google Scholar]
- Tavares C., Sakata R. Caffeine in the Treatment of Pain. Braz. J. Anesthesiol. 2012;62:387–401. doi: 10.1016/S0034-7094(12)70139-3. [PubMed] [CrossRef] [Google Scholar]
- Fredholm B.B. Adenosine, Adenosine Receptors and the Actions of Caffeine. Pharmacol. Toxicol. 1995;76:93–101. doi: 10.1111/j.1600-0773.1995.tb00111.x. [PubMed] [CrossRef] [Google Scholar]
- Arnaud M.J. Methylxanthines. Springer; Berlin/Heidelberg, Germany: 2010. Pharmacokinetics and Metabolism of Natural Methylxanthines in Animal and Man; pp. 33–91. [PubMed] [Google Scholar]
- Pickering C., Kiely J. Are the Current Guidelines on Caffeine Use in Sport Optimal for Everyone? Inter-individual Variation in Caffeine Ergogenicity, and a Move Towards Personalised Sports Nutrition. Sports Med. 2018;48:7–16. doi: 10.1007/s40279-017-0776-1. [PMC free article] [PubMed] [CrossRef] [Google Scholar]
- Sachse C., Brockmöller J., Bauer S., Roots I. Functional significance of a C?A polymorphism in intron 1 of the cytochrome P450 CYP1A2 gene tested with caffeine. Br. J. Clin. Pharmacol. 1999;47:445–449. doi: 10.1046/j.1365-2125.1999.00898.x. [PMC free article] [PubMed] [CrossRef] [Google Scholar]
- Womack C.J., Saunders M.J., Bechtel M.K., Bolton D.J., Martin M., Luden N.D., Dunham W., Hancock M. The influence of a CYP1A2 polymorphism on the ergogenic effects of caffeine. J. Int. Soc. Sports Nutr. 2012;9:7. doi: 10.1186/1550-2783-9-7. [PMC free article] [PubMed] [CrossRef] [Google Scholar]
- Pataky M.W., Womack C.J., Saunders M.J., Goffe J.L., D’Lugos A.C., Elsohemy A., Luden N.D. Caffeine and 3-km cycling performance: Effects of mouth rinsing, genotype, and time of day. Scand. J. Med. Sci. Sports. 2015;26:613–619. doi: 10.1111/sms.12501. [PubMed] [CrossRef] [Google Scholar]
- Algrain H.A., Thomas R.M., Carrillo A.E., Ryan E.J., Kim C.-H., Lettan R.B., Ryan E.J. The Effects of a Polymorphism in the Cytochrome P450 CYP1A2 Gene on Performance Enhancement with Caffeine in Recreational Cyclists. J. Caffeine Res. 2016;6:34–39. doi: 10.1089/jcr.2015.0029. [CrossRef] [Google Scholar]
- Klein C.S., Clawson A., Martin M., Saunders M.J., Flohr J.A., Bechtel M.K., Dunham W., Hancock M., Womack C.J. The Effect of Caffeine on Performance in Collegiate Tennis Players. J. Caffeine Res. 2012;2:111–116. doi: 10.1089/jcr.2012.0019. [CrossRef] [Google Scholar]
- Salinero J.J., Lara B., Ruiz-Vicente D., Areces F., Puente-Torres C., Gallo-Salazar C., Pascual T., Del Coso J. CYP1A2 Genotype Variations Do Not Modify the Benefits and Drawbacks of Caffeine during Exercise: A Pilot Study. Nutrients. 2017;9:269. doi: 10.3390/nu9030269. [PMC free article] [PubMed] [CrossRef] [Google Scholar]
- Nehlig A. Interindividual Differences in Caffeine Metabolism and Factors Driving Caffeine Consumption. Pharmacol. Rev. 2018;70:384–411. doi: 10.1124/pr.117.014407. [PubMed] [CrossRef] [Google Scholar]
- Carswell A.T., Howland K., Martinez-Gonzalez B., Baron P., Davison G. The effect of caffeine on cognitive performance is influenced by CYP1A2 but not ADORA2A genotype, yet neither genotype affects exercise performance in healthy adults. Graefe’s Arch. Clin. Exp. Ophthalmol. 2020;120:1495–1508. doi: 10.1007/s00421-020-04384-8. [PMC free article] [PubMed] [CrossRef] [Google Scholar]
- Loy B.D., O’Connor P.J., Lindheimer J.B., Covert S.F. Caffeine Is Ergogenic for Adenosine A2A Receptor Gene (ADORA2A) T Allele Homozygotes: A Pilot Study. J. Caffeine Res. 2015;5:73–81. doi: 10.1089/jcr.2014.0035. [CrossRef] [Google Scholar]
- Ribeiro J.A., Sebastio A.M. Caffeine and adenosine. J. Alzheimer’s Dis. 2010;20:S3–S15. doi: 10.3233/JAD-2010-1379. [PubMed] [CrossRef] [Google Scholar]
- McLellan T.M., Caldwell J.A., Lieberman H.R. A review of caffeine’s effects on cognitive, physical and occupational performance. Neurosci. Biobehav. Rev. 2016;71:294–312. doi: 10.1016/j.neubiorev.2016.09.001. [PubMed] [CrossRef] [Google Scholar]
- Kong H., Jones P.P., Koop A., Zhang L., Duff H.J., Chen S.R.W. Caffeine induces Ca2+ release by reducing the threshold for luminal Ca2+ activation of the ryanodine receptor. Biochem. J. 2008;414:441–452. doi: 10.1042/BJ20080489. [PMC free article] [PubMed] [CrossRef] [Google Scholar]
- Yang J.Y., Yang G., Ren J., Zhao J., Li S. Caffeine Suppresses GABA Receptor-Mediated Current in Rat Primary Sensory Neurons via Inhibition of Intracellular Phosphodiesterase. Neurophysiology. 2015;47:108–114. doi: 10.1007/s11062-015-9506-1. [CrossRef] [Google Scholar]
- Paiva C.L.R.S., Beserra B.T.S., Reis C.E.G., Dorea J.G., Da Costa T.H.M., Amato A.A. Consumption of coffee or caffeine and serum concentration of inflammatory markers: A systematic review. Crit. Rev. Food Sci. Nutr. 2019;59:652–663. doi: 10.1080/10408398.2017.1386159. [PubMed] [CrossRef] [Google Scholar]
- Zampelas A., Panagiotakos D.B., Pitsavos C., Chrysohoou C., Stefanadis C. Associations between coffee consumption and inflammatory markers in healthy persons: The ATTICA study. Am. J. Clin. Nutr. 2004;80:862–867. doi: 10.1093/ajcn/80.4.862. [PubMed] [CrossRef] [Google Scholar]
- EFSA Panel on Dietetic Products, Nutrition and Allergies (NDA) Scientific Opinion on the safety of caffeine. EFSA J. 2015;13 doi: 10.2903/j.efsa.2015.4102. [CrossRef] [Google Scholar]
- Musgrave I.F., Farrington R.L., Hoban C., Byard R.W. Caffeine toxicity in forensic practice: Possible effects and under-appreciated sources. Forensic Sci. Med. Pathol. 2016;12:299–303. doi: 10.1007/s12024-016-9786-9. [PubMed] [CrossRef] [Google Scholar]
- Boison D. Methylxanthines, seizures, and excitotoxicity. Handb. Exp. Pharmacol. 2011;200:251–266. [PMC free article] [PubMed] [Google Scholar]
- Nawrot P., Jordan S., Eastwood J., Rotstein J., Hugenholtz A., Feeley M. Effects of caffeine on human health. Food Addit. Contam. 2003;20:1–30. doi: 10.1080/0265203021000007840. [PubMed] [CrossRef] [Google Scholar]
- Seifert S.M., Schaechter J.L., Hershorin E.R., Lipshultz S.E. Health Effects of Energy Drinks on Children, Adolescents, and Young Adults. Pediatrics. 2011;127:511–528. doi: 10.1542/peds.2009-3592. [PMC free article] [PubMed] [CrossRef] [Google Scholar]
- Rebola N., Rodrigues R.J., Oliveira C.R., Cunha R.A. Different roles of adenosine A1, A2A and A3 receptors in controlling kainate-induced toxicity in cortical cultured neurons. Neurochem. Int. 2005;47:317–325. doi: 10.1016/j.neuint.2005.05.009. [PubMed] [CrossRef] [Google Scholar]
- Cunha R. Adenosine as a neuromodulator and as a homeostatic regulator in the nervous system: Different roles, different sources and different receptors. Neurochem. Int. 2001;38:107–125. doi: 10.1016/S0197-0186(00)00034-6. [PubMed] [CrossRef] [Google Scholar]
- Haskó G., Pacher P., Deitch E.A., Vizi E.S. Shaping of monocyte and macrophage function by adenosine receptors. Pharmacol. Ther. 2007;113:264–275. doi: 10.1016/j.pharmthera.2006.08.003. [PMC free article] [PubMed] [CrossRef] [Google Scholar]
- Fredholm B.B., Arslan G., Halldner L., Kull B., Schulte G., Wasserman W. Structure and function of adenosine receptors and their genes. Naunyn-Schmiedeberg’s Arch. Pharmacol. 2000;362:364–374. doi: 10.1007/s002100000313. [PubMed] [CrossRef] [Google Scholar]
- Morelli M., Carta A.R., Kachroo A., Schwarzschild M.A. Pathophysiological roles for purines. Adenosine, caffeine and urate. Prog. Brain Res. 2010;183:183–208. [PMC free article] [PubMed] [Google Scholar]
- Polito C.A., Cai Z.-Y., Shi Y.-L., Li X.-M., Yang R., Shi M., Li Q.-S., Ma S.-C., Xiang L.-P., Wang K.-R., et al. Association of Tea Consumption with Risk of Alzheimer’s Disease and Anti-Beta-Amyloid Effects of Tea. Nutrients. 2018;10:655. doi: 10.3390/nu10050655. [PMC free article] [PubMed] [CrossRef] [Google Scholar]
- Kaplan G.B., Greenblatt D.J., Ehrenberg B.L., Goddard J.E., Bs M.M.C., Ba J.S.H., Shader R. Dose-Dependent Pharmacokinetics and Psychomotor Effects of Caffeine in Humans. J. Clin. Pharmacol. 1997;37:693–703. doi: 10.1002/j.1552-4604.1997.tb04356.x. [PubMed] [CrossRef] [Google Scholar]
- Watson E.J., Coates A.M., Kohler M., Banks S. Caffeine Consumption and Sleep Quality in Australian Adults. Nutrients. 2016;8:479. doi: 10.3390/nu8080479. [PMC free article] [PubMed] [CrossRef] [Google Scholar]
- Watson E.J., Banks S., Coates A.M., Kohler M.J. The Relationship between Caffeine, Sleep, and Behavior in Children. J. Clin. Sleep Med. 2017;13:533–543. doi: 10.5664/jcsm.6536. [PMC free article] [PubMed] [CrossRef] [Google Scholar]
- Richards G., Smith A. Caffeine consumption and self-assessed stress, anxiety, and depression in secondary school children. J. Psychopharmacol. 2015;29:1236–1247. doi: 10.1177/0269881115612404. [PMC free article] [PubMed] [CrossRef] [Google Scholar]
- Dando R., Dvoryanchikov G., Pereira E., Chaudhari N., Roper S.D. Adenosine Enhances Sweet Taste through A2B Receptors in the Taste Bud. J. Neurosci. 2012;32:322–330. doi: 10.1523/JNEUROSCI.4070-11.2012. [PMC free article] [PubMed] [CrossRef] [Google Scholar]
- Holtzman S.G., Mante S., Minneman K.P. Role of adenosine receptors in caffeine tolerance. J. Pharmacol. Exp. Ther. 1991;256:62–68. [PubMed] [Google Scholar]
- Choo E., Picket B., Dando R. Caffeine May Reduce Perceived Sweet Taste in Humans, Supporting Evidence That Adenosine Receptors Modulate Taste. J. Food Sci. 2017;82:2177–2182. doi: 10.1111/1750-3841.13836. [PubMed] [CrossRef] [Google Scholar]
- Pickering C. Are caffeine’s performance-enhancing effects partially driven by its bitter taste? Med. Hypotheses. 2019;131:109301. doi: 10.1016/j.mehy.2019.109301. [PubMed] [CrossRef] [Google Scholar]
- Gramling L., Kapoulea E., Murphy C. Taste Perception and Caffeine Consumption: An fMRI Study. Nutrients. 2018;11:34. doi: 10.3390/nu11010034. [PMC free article] [PubMed] [CrossRef] [Google Scholar]
- Lashley T., Schott J.M., Weston P., Murray C.E., Wellington H., Keshavan A., Foti S.C., Foiani M., Toombs J., Rohrer J.D., et al. Molecular biomarkers of Alzheimer’s disease: Progress and prospects. Dis. Model. Mech. 2018;11 doi: 10.1242/dmm.031781. [PMC free article] [PubMed] [CrossRef] [Google Scholar]
- Li S., Geiger N.H., Soliman M.L., Hui L., Geiger J.D., Chen X. Caffeine, Through Adenosine A3 Receptor-Mediated Actions, Suppresses Amyloid-? Protein Precursor Internalization and Amyloid-? Generation. J. Alzheimer’s Dis. 2015;47:73–83. doi: 10.3233/JAD-142223. [PMC free article] [PubMed] [CrossRef] [Google Scholar]
- Rahman A. The Role of Adenosine in Alzheimers Disease. Curr. Neuropharmacol. 2009;7:207–216. doi: 10.2174/157015909789152119. [PMC free article] [PubMed] [CrossRef] [Google Scholar]
- Cunha R.A. Neuroprotection by adenosine in the brain: From A1 receptor activation to A2A receptor blockade. Purinergic Signal. 2005;1:111–134. doi: 10.1007/s11302-005-0649-1. [PMC free article] [PubMed] [CrossRef] [Google Scholar]
- Kaster M.P., Machado N.J., Silva H.B., Nunes A.M., Ardais A.P., Santana M., Baqi Y., Müller C.E., Rodrigues A.L., Porciúncula L.O., et al. Caffeine acts through neuronal adenosine A2A receptors to prevent mood and memory dysfunction triggered by chronic stress. Proc. Natl. Acad. Sci. USA. 2015;112:7833–7838. doi: 10.1073/pnas.1423088112. [PMC free article] [PubMed] [CrossRef] [Google Scholar]
- Maia L., De Mendonca A. Does caffeine intake protect from Alzheimer’s disease? Eur. J. Neurol. 2002;9:377–382. doi: 10.1046/j.1468-1331.2002.00421.x. [PubMed] [CrossRef] [Google Scholar]
- Ritchie K., Carriere I., de Mendonça A., Portet F., Dartigues J.-F., Rouaud O., Barberger-Gateau P., Ancelin M.-L. The neuroprotective effects of caffeine: A prospective population study (the Three City Study) Neurology. 2007;69:536–545. doi: 10.1212/01.wnl.0000266670.35219.0c. [PubMed] [CrossRef] [Google Scholar]
- Eskelinen M.H., Ngandu T., Tuomilehto J., Soininen H., Kivipelto M. Midlife Coffee and Tea Drinking and the Risk of Late-Life Dementia: A Population-Based CAIDE Study. J. Alzheimer’s Dis. 2009;16:85–91. doi: 10.3233/JAD-2009-0920. [PubMed] [CrossRef] [Google Scholar]
- Lindsay J., Laurin D., Verreault R., Hébert R., Helliwell B., Hill G.B., McDowell I. Risk Factors for Alzheimer’s Disease: A Prospective Analysis from the Canadian Study of Health and Aging. Am. J. Epidemiol. 2002;156:445–453. doi: 10.1093/aje/kwf074. [PubMed] [CrossRef] [Google Scholar]
- Dong X., Li S., Sun J., Li Y., Zhang D. Association of Coffee, Decaffeinated Coffee and Caffeine Intake from Coffee with Cognitive Performance in Older Adults: National Health and Nutrition Examination Survey (NHANES) 2011–2014. Nutrients. 2020;12:840. doi: 10.3390/nu12030840. [PMC free article] [PubMed] [CrossRef] [Google Scholar]
- Camfield D.A., Silber B.Y., Scholey A.B., Nolidin K., Goh A., Stough C. A Randomised Placebo-Controlled Trial to Differentiate the Acute Cognitive and Mood Effects of Chlorogenic Acid from Decaffeinated Coffee. PLoS ONE. 2013;8:e82897. doi: 10.1371/journal.pone.0082897. [PMC free article] [PubMed] [CrossRef] [Google Scholar]
- Haskell-Ramsay C.F., Jackson P.A., Forster J.S., Dodd F.L., Bowerbank S.L., Kennedy D.O. The Acute Effects of Caffeinated Black Coffee on Cognition and Mood in Healthy Young and Older Adults. Nutrients. 2018;10:1386. doi: 10.3390/nu10101386. [PMC free article] [PubMed] [CrossRef] [Google Scholar]
- Fujimaki M., Saiki S., Li Y., Kaga N., Taka H., Hatano T., Ishikawa K.-I., Oji Y., Mori A., Okuzumi A., et al. Serum caffeine and metabolites are reliable biomarkers of early Parkinson disease. Neurology. 2018;90:e404–e411. doi: 10.1212/WNL.0000000000004888. [PMC free article] [PubMed] [CrossRef] [Google Scholar]
- Ross G.W., Abbott R.D., Petrovitch H., Morens D.M., Grandinetti A., Tung K.-H., Tanner C.M., Masaki K.H., Blanchette P.L., Curb J.D., et al. Association of Coffee and Caffeine Intake With the Risk of Parkinson Disease. JAMA. 2000;283:2674–2679. doi: 10.1001/jama.283.20.2674. [PubMed] [CrossRef] [Google Scholar]
- Liu R., Guo X., Park Y., Huang X., Sinha R., Freedman N.D., Hollenbeck A.R., Blair A., Chen H. Caffeine Intake, Smoking, and Risk of Parkinson Disease in Men and Women. Am. J. Epidemiol. 2012;175:1200–1207. doi: 10.1093/aje/kwr451. [PMC free article] [PubMed] [CrossRef] [Google Scholar]
- Palacios N., Gao X., ScD M.L.M., Schwarzschild M.A., Shah R., Gapstur S., Ascherio A. Caffeine and risk of Parkinson’s disease in a large cohort of men and women. Mov. Disord. 2012;27:1276–1282. doi: 10.1002/mds.25076. [PMC free article] [PubMed] [CrossRef] [Google Scholar]
- Postuma R.B., Anang J., Pelletier A., Joseph L., Moscovich M., Grimes D., Furtado S., Munhoz R.P., Appel-Cresswell S., Moro A., et al. Caffeine as symptomatic treatment for Parkinson disease (Café-PD) Neurology. 2017;89:1795–1803. doi: 10.1212/WNL.0000000000004568. [PMC free article] [PubMed] [CrossRef] [Google Scholar]
- Roshan M.H.K., Tambo A., Pace N.P. Potential Role of Caffeine in the Treatment of Parkinson’s Disease. Open Neurol. J. 2016;10:42–58. doi: 10.2174/1874205X01610010042. [PMC free article] [PubMed] [CrossRef] [Google Scholar]
- Simon D.K., Wu C., Tilley B.C., Wills A.-M., Aminoff M.J., Bainbridge J., Hauser R.A., Schneider J.S., Sharma S., Singer C., et al. Caffeine and Progression of Parkinson Disease: A deleterious interaction with creatine. Clin. Neuropharmacol. 2015;38:163–169. doi: 10.1097/WNF.0000000000000102. [PMC free article] [PubMed] [CrossRef] [Google Scholar]
- Hespel P., Eijnde B.O., Van Leemputte M. Opposite actions of caffeine and creatine on muscle relaxation time in humans. J. Appl. Physiol. 2002;92:513–518. doi: 10.1152/japplphysiol.00255.2001. [PubMed] [CrossRef] [Google Scholar]
- Vandenberghe K., Gillis N., Van Leemputte M., Van Hecke P., Vanstapel F., Hespel P. Caffeine counteracts the ergogenic action of muscle creatine loading. J. Appl. Physiol. 1996;80:452–457. doi: 10.1152/jappl.1996.80.2.452. [PubMed] [CrossRef] [Google Scholar]
- Simonin C., Duru C., Salleron J., Hincker P., Charles P., Delval A., Youssov K., Burnouf S., Azulay J.-P., Verny C., et al. Association between caffeine intake and age at onset in Huntington’s disease. Neurobiol. Dis. 2013;58:179–182. doi: 10.1016/j.nbd.2013.05.013. [PubMed] [CrossRef] [Google Scholar]
- MacDonald M.E., Ambrose C.M., Duyao M.P., Myers R.H., Lin C., Srinidhi L., Barnes G., Taylor S.A., James M., Groot N., et al. A novel gene containing a trinucleotide repeat that is expanded and unstable on Huntington’s disease chromosomes. Cell. 1993;72:971–983. doi: 10.1016/0092-8674(93)90585-E. [PubMed] [CrossRef] [Google Scholar]
- Blum D., Chern Y., Domenici M.R., Buee L., Lin C.-Y., Rea W., Ferré S., Popoli P. The Role of Adenosine Tone and Adenosine Receptors in Huntington’s Disease. J. Caffeine Adenosine Res. 2018;8:43–58. doi: 10.1089/caff.2018.0006. [PMC free article] [PubMed] [CrossRef] [Google Scholar]
- Zuccato C., Valenza M., Cattaneo E. Molecular Mechanisms and Potential Therapeutical Targets in Huntington’s Disease. Physiol. Rev. 2010;90:905–981. doi: 10.1152/physrev.00041.2009. [PubMed] [CrossRef] [Google Scholar]
- Stack E.C., Dedeoglu A., Smith K.M., Cormier K., Kubilus J.K., Bogdanov M., Matson W.R., Yang L., Jenkins B.G., Luthi-Carter R., et al. Neuroprotective Effects of Synaptic Modulation in Huntington’s Disease R6/2 Mice. J. Neurosci. 2007;27:12908–12915. doi: 10.1523/JNEUROSCI.4318-07.2007. [PMC free article] [PubMed] [CrossRef] [Google Scholar]
- Schwarzschild M.A., Agnati L., Fuxe K., Chen J.-F., Morelli M. Targeting adenosine A2A receptors in Parkinson’s disease. Trends Neurosci. 2006;29:647–654. doi: 10.1016/j.tins.2006.09.004. [PubMed] [CrossRef] [Google Scholar]
- Blum D., Hourez R., Galas M.-C., Popoli P., Schiffmann S.N. Adenosine receptors and Huntington’s disease: Implications for pathogenesis and therapeutics. Lancet Neurol. 2003;2:366–374. doi: 10.1016/S1474-4422(03)00411-3. [PubMed] [CrossRef] [Google Scholar]
- Tanner C., Marder K., Eberly S., Biglan K., Oakes D., Shoulson I. Selected health and lifestyle factors, cytosine-adenine-guanine status, and phenoconversion in Huntington’s disease. Mov. Disord. 2018;33:472–478. doi: 10.1002/mds.27239. [PMC free article] [PubMed] [CrossRef] [Google Scholar]
- Temple J.L., Bernard C., Lipshultz S.E., Czachor J.D., Westphal J.A., Mestre M.A. The Safety of Ingested Caffeine: A Comprehensive Review. Front. Psychiatry. 2017;8:80. doi: 10.3389/fpsyt.2017.00080. [PMC free article] [PubMed] [CrossRef] [Google Scholar]
- Overstreet D.S., Penn T.M., Cable S.T., Aroke E.N., Goodin B.R. Higher habitual dietary caffeine consumption is related to lower experimental pain sensitivity in a community-based sample. Psychopharmacology. 2018;235:3167–3176. doi: 10.1007/s00213-018-5016-3. [PMC free article] [PubMed] [CrossRef] [Google Scholar]
- Sawynok J. Adenosine receptor activation and nociception. Eur. J. Pharmacol. 1998;347:1–11. doi: 10.1016/S0014-2999(97)01605-1. [PubMed] [CrossRef] [Google Scholar]
- Sawynok J., Liu X.J. Adenosine in the spinal cord and periphery: Release and regulation of pain. Prog. Neurobiol. 2003;69:313–340. doi: 10.1016/S0301-0082(03)00050-9. [PubMed] [CrossRef] [Google Scholar]
- Latini S., Pedata F. Adenosine in the central nervous system: Release mechanisms and extracellular concentrations. J. Neurochem. 2001;79:463–484. doi: 10.1046/j.1471-4159.2001.00607.x. [PubMed] [CrossRef] [Google Scholar]
- Rogers N.L., Dinges D.F. Caffeine: Implications for Alertness in Athletes. Clin. Sports Med. 2005;24:e1–e13. doi: 10.1016/j.csm.2004.12.012. [PubMed] [CrossRef] [Google Scholar]
- Sollevi A. Adenosine for pain control. Acta Anaesthesiol. Scand. 1997;41:135–136. doi: 10.1111/j.1399-6576.1997.tb05532.x. [PubMed] [CrossRef] [Google Scholar]
- Baratloo A., Rouhipour A., Forouzanfar M.M., Safari S., Amiri M., Negida A. The Role of Caffeine in Pain Management: A Brief Literature Review. Anesthesiol. Pain Med. 2016;6:e33193. doi: 10.5812/aapm.33193. [PMC free article] [PubMed] [CrossRef] [Google Scholar]
- Derry C.J., Derry S., Moore R.A. Caffeine as an analgesic adjuvant for acute pain in adults. Cochrane Database Syst. Rev. 2014;2014:CD009281. doi: 10.1002/14651858.CD009281.pub3. [PMC free article] [PubMed] [CrossRef] [Google Scholar]
- Nikolajsen L., Haroutiunian S. Koffein som adjuverende analgetikum til behandling af akutte smerter-en gennemgang af et Cochranereview. Ugeskr Læger. 2013;175:2486–2488. [PubMed] [Google Scholar]
- Zwilling M., Theiss C., Matschke V. Caffeine and NAD+Improve Motor Neural Integrity of Dissociated Wobbler Cells in Vitro. Antioxidants. 2020;9:460. doi: 10.3390/antiox9060460. [PMC free article] [PubMed] [CrossRef] [Google Scholar]
- Lucas M., O’Reilly E.J., Pan A., Mirzaei F., Willett W.C., Okereke O.I., Ascherio A. Coffee, caffeine, and risk of completed suicide: Results from three prospective cohorts of American adults. World J. Biol. Psychiatry. 2014;15:377–386. doi: 10.3109/15622975.2013.795243. [PMC free article] [PubMed] [CrossRef] [Google Scholar]
- Botella P., Parra A. Coffee increases state anxiety in males but not in females. Hum. Psychopharmacol. Clin. Exp. 2003;18:141–143. doi: 10.1002/hup.444. [PubMed] [CrossRef] [Google Scholar]
- Iranpour S., Sabour S. Inverse association between caffeine intake and depressive symptoms in US adults: Data from National Health and Nutrition Examination Survey (NHANES) 2005–2006. Psychiatry Res. 2019;271:732–739. doi: 10.1016/j.psychres.2018.11.004. [PubMed] [CrossRef] [Google Scholar]
- Kendler K.S., Myers J., Gardner C.O. Caffeine intake, toxicity and dependence and lifetime risk for psychiatric and substance use disorders: An epidemiologic and co-twin control analysis. Psychol. Med. 2006;36:1717–1725. doi: 10.1017/S0033291706008622. [PubMed] [CrossRef] [Google Scholar]
- Bertasi R.A.O., Humeda Y., Bertasi T.G.O., Zins Z., Kimsey J., Pujalte G. Caffeine Intake and Mental Health in College Students. Cureus. 2021;13 doi: 10.7759/cureus.14313. [PMC free article] [PubMed] [CrossRef] [Google Scholar]
- Hedström A.K., Mowry E.M., Gianfrancesco M.A., Shao X.A., Schaefer C., Shen L., Olsson T., Barcellos L.F., Alfredsson L. High consumption of coffee is associated with decreased multiple sclerosis risk; results from two independent studies. J. Neurol. Neurosurg. Psychiatry. 2016;87:454–460. doi: 10.1136/jnnp-2015-312176. [PMC free article] [PubMed] [CrossRef] [Google Scholar]
- Konishi Y., Hori H., Ide K., Katsuki A., Atake K., Igata R., Kubo T., Tominaga H., Beppu H., Asahara T., et al. Effect of single caffeine intake on neuropsychological functions in healthy volunteers: A double-blind placebo-controlled study. PLoS ONE. 2018;13:e0202247. doi: 10.1371/journal.pone.0202247. [PMC free article] [PubMed] [CrossRef] [Google Scholar]
- Yu N.Y., Bieder A., Raman A., Mileti E., Katayama S., Einarsdottir E., Fredholm B.B., Falk A., Tapia-Páez I., Daub C.O., et al. Acute doses of caffeine shift nervous system cell expression profiles toward promotion of neuronal projection growth. Sci. Rep. 2017;7:11458. doi: 10.1038/s41598-017-11574-6. [PMC free article] [PubMed] [CrossRef] [Google Scholar]
- Kawachi I., Willett W.C., Colditz G.A., Stampfer M.J., Speizer F.E. A prospective study of coffee drinking and suicide in women. Arch. Intern. Med. 1996;156:521–525. doi: 10.1001/archinte.1996.00440050067008. [PubMed] [CrossRef] [Google Scholar]
- Cappelletti S., Piacentino D., Fineschi V., Frati P., Cipolloni L., Aromatario M. Caffeine-Related Deaths: Manner of Deaths and Categories at Risk. Nutrients. 2018;10:611. doi: 10.3390/nu10050611. [PMC free article] [PubMed] [CrossRef] [Google Scholar]
- Tanskanen A., Tuomilehto J., Viinamäki H., Vartiainen E., Lehtonen J., Puska P. Heavy coffee drinking and the risk of suicide. Eur. J. Epidemiol. 2000;16:789–791. doi: 10.1023/A:1007614714579. [PubMed] [CrossRef] [Google Scholar]
- Lucas M., Mirzaei F., Pan A., Okereke O.I., Willett W.C., O’Reilly É.J., Koenen K., Ascherio A. Coffee, Caffeine, and Risk of Depression Among Women. Arch. Intern. Med. 2011;171:1571–1578. doi: 10.1001/archinternmed.2011.393. [PMC free article] [PubMed] [CrossRef] [Google Scholar]
- Tomar N., De R.K. Immunoinformatics. Volume 1184. Humana Press; New York, NY, USA: 2014. A Brief Outline of the Immune System; pp. 3–12. [PubMed] [CrossRef] [Google Scholar]
- Venter C., Eyerich S., Sarin T., Klatt K.C. Nutrition and the Immune System: A Complicated Tango. Nutrients. 2020;12:818. doi: 10.3390/nu12030818. [PMC free article] [PubMed] [CrossRef] [Google Scholar]
- Gibbs B.F., Silva I.G., Prokhorov A., Abooali M., Yasinska I., A Casely-Hayford M., Berger S.M., Fasler-Kan E., Sumbayev V.V. Caffeine affects the biological responses of human hematopoietic cells of myeloid lineage via downregulation of the mTOR pathway and xanthine oxidase activity. Oncotarget. 2015;6:28678–28692. doi: 10.18632/oncotarget.5212. [PMC free article] [PubMed] [CrossRef] [Google Scholar]
- Chavez-Valdez R., Wills-Karp M., Ahlawat R., Cristofalo E.A., Nathan A., Gauda E.B. Caffeine Modulates TNF-? Production by Cord Blood Monocytes: The Role of Adenosine Receptors. Pediatr. Res. 2009;65:203–208. doi: 10.1203/PDR.0b013e31818d66b1. [PubMed] [CrossRef] [Google Scholar]
- Chavez-Valdez R., Ahlawat R., Wills-Karp M., Gauda E.B. Mechanisms of modulation of cytokine release by human cord blood monocytes exposed to high concentrations of caffeine. Pediatr. Res. 2016;80:101–109. doi: 10.1038/pr.2016.50. [PMC free article] [PubMed] [CrossRef] [Google Scholar]
- Steck R.P., Hill S.L., Weagel E., Weber S., Robison R.A., O’Neill K.L. Pharmacologic immunosuppression of mononuclear phagocyte phagocytosis by caffeine. Pharmacol. Res. Perspect. 2015;3:e00180. doi: 10.1002/prp2.180. [PMC free article] [PubMed] [CrossRef] [Google Scholar]
- Shushtari N., Froushani S.M.A. Caffeine Augments the Instruction of Anti-Inflammatory Macrophages by the Conditioned Medium of Mesenchymal Stem Cells. Cell J. 2017;19:415–424. doi: 10.22074/CELLJ.2017.4364. [PMC free article] [PubMed] [CrossRef] [Google Scholar]
- Bojarowicz H., Przygoda M. Powszechno?? stosowania kofeiny oraz jej dzia?anie na organizm Caffeine. Part, I. Common use of caffeine and its effect on human organism. Probl. Hig. Epidemiol. 2012;93:8–13. [Google Scholar]
- Lohsiriwat S., Puengna N., Leelakusolvong S. Effect of caffeine on lower esophageal sphincter pressure in Thai healthy volunteers. Dis. Esophagus. 2006;19:183–188. doi: 10.1111/j.1442-2050.2006.00562.x.